We did not act early enough on climate change, and we continue to burn through our carbon budget too fast. As a result, we are faced with the need to capture and store away increasingly large amounts of carbon dioxide (CO2). Trees are great at this but they can sequester at most a fraction of what’s needed. The International Energy Agency has estimated that we will need to capture 1.6 billion tons of CO2 by 2030 if we are to meet our climate goals, and five times that by 2050. Our plan is to bury it deep inside the planet. (1) Many people think that is pretty straight-forward, but do we know how to do it and can we do it fast enough?
We have been burying CO2 on a small scale for many years. The fossil fuel industry has been injecting CO2 into some of its less productive oil wells and coal mines in an effort to extract more oil and methane. As shown in the picture below, carbon dioxide can also be stored in saline formations, large aquifers filled with brine in sedimentary rock, that are deep enough to accommodate pressurized CO2. (2)
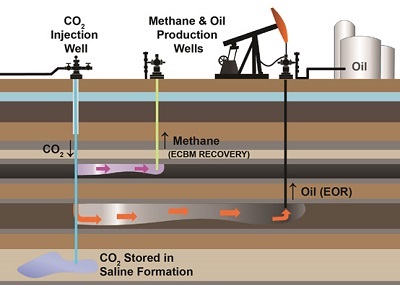
CO2 has been injected into coal beds and oil fields to help push out extra methane and oil. It has also been injected into saline reservoirs for storage. Source: National Energy Technology Laboratory
It turns out that we have a lot of these sedimentary basins in the US, both under land and off the coast. There appears to be more than enough room to store all of the CO2 we would ever need to store.
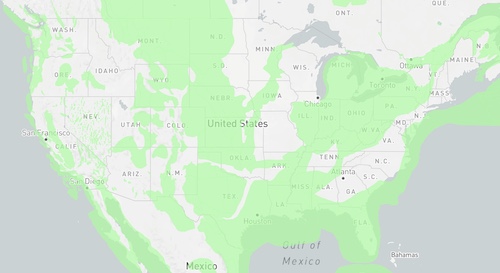
Sedimentary basins are common beneath the continental United States. Source: Department of Energy’s Carbon Storage Atlas (NATCARB)
How is carbon dioxide stored in one of these basins? It is compressed and injected into the earth, typically at a depth of one or more kilometers. Once down there, it spreads out through pores in the sedimentary rock (e.g., sandstone or limestone) and is trapped by a (hopefully) impermeable “capstone” above the aquifer (e.g., slate), or possibly by faults in the rock, as shown below.
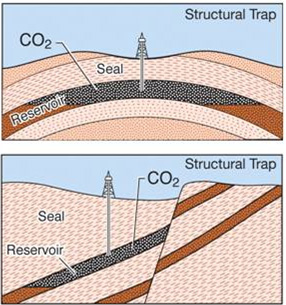
Carbon dioxide (gray) seeps through sedimentary pores (dark brown) before being trapped by a capstone (light brown, top) and/or shifted impermeable layers (bottom). Source: National Energy Technology Laboratory
Over the years as the carbon dioxide stays down there, some of it becomes trapped in the pores of the rocks, where it is less likely to leak. Some of it dissolves into the saline brine. And some of it reacts with the walls of the cavities to form minerals. (3)
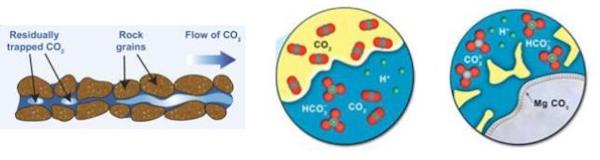
Carbon dioxide becomes trapped in rock pores (left), dissolved in brine (middle), and precipitated in minerals such as magnesium carbonate (right) over time. Source: National Energy Technology Laboratory
We have a fossil-trained workforce with the skills needed to evaluate the aquifers, build necessary pipelines, and drill, maintain, and monitor the wells. The cost of basic carbon storage is manageable, around $10-$20/ton, which is generally much less than the cost of capturing and transporting it. So this all seems pretty promising, right?
The crux is that we have to scale up very, very quickly. This year we are capturing about 40 Mt of CO2. We need to scale that by 40x in the next eight years to align with a pathway to net-zero by 2050. That is a massive acceleration from what at this point is still in many ways a science experiment.
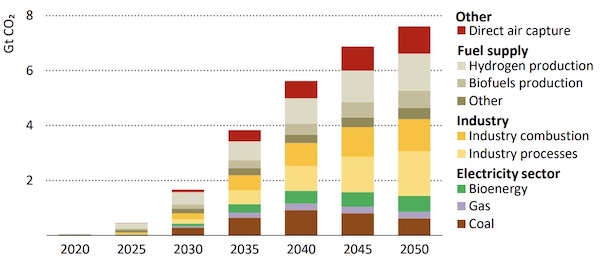
To achieve net-zero, IEA estimates we need to capture this amount of CO2 globally, per source. Source: International Energy Agency Net Zero Roadmap
There is much that we don’t understand about injecting into these aquifers, and in particular injecting quickly and safely. For example, the products of the reactions that occur when the CO2 mixes with the brine and the rocks can impede the injection itself. Minerals that form can clog the openings in the porous rock and precipitate can coat the rock surfaces and make them less reactive. A large aquifer can become pretty small and/or riddled with wells if we cannot figure out how to reduce these effects so the CO2 can disperse effectively.
There are risks of contaminating our drinking water. The CO2 injections can push saltier water upwards towards the drinking water. Or the CO2 itself can mix into the drinking water, acidifying it and leaching toxins from the rock. How do we detect and prevent these issues deep underground, especially when we are developing 100+ new sites every year?
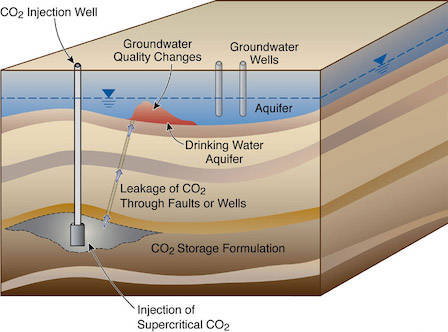
Carbon dioxide can leak up through faults or along the casing of a well to acidify the drinking water supply. Image source: Berkeley Lab
You also get the potential for earthquakes when you are putting such a large volume of fluid deep into the Earth. We have seen in places as far flung as Oklahoma, Arkansas, Illinois, and West Texas that even a very small increase in pressure in some sedimentary aquifers can lead to substantial earthquakes. The oil and gas companies have seen this when they dispose of wastewater.
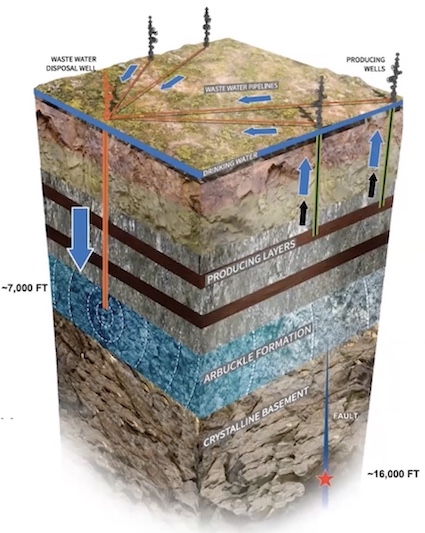
Wastewater from productive wells in Oklahoma was injected into an aquifer 7000 feet below the surface. The slight resulting increase in pressure in this aquifer was transmitted through a fault in the crystalline basement below, leading to noticeable earthquakes. Source: Stanford Center for Carbon Storage webinar by Geophysicist and Stanford Professor Emeritus Mark Zoback
Slight pressure increases can be transmitted long distances to deeper and more brittle layers, which can result in larger tremors. In Oklahoma, earthquakes occurred at a depth of 6-7 km, far below where the wastewater was injected. The largest quake registered at 5.8. These tapered off only when wastewater injection was curtailed.
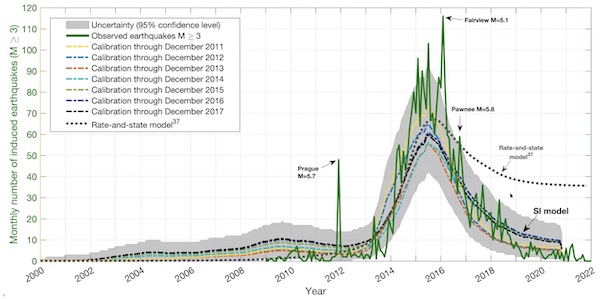
Earthquakes in northern Oklahoma, shown in green, decreased when less water was injected. Source: Stanford Center for Carbon Storage webinar by Geophysicist and Stanford Professor Emeritus Mark Zoback
West Texas is seeing an effect very much like this right now. Earlier this year the Texas regulator of oil and gas wells moved to suspend deep well water disposal in an attempt to mitigate the earthquakes, but they continue to happen, as shown in the snapshot below from a few days ago.
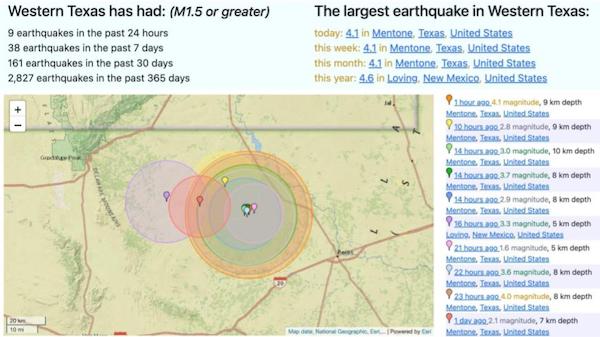
Source: https://earthquaketrack.com/r/western-texas/recent, April 28, 2022
It is possible to manage the pressure in these aquifers by extracting some of the brine, but processing and managing that brine is costly, and we don’t know how to do it well yet. Alternatively, it is possible to identify and bypass those aquifers with the most potential to cause fault slips. Geophysicist and Stanford Professor Emeritus Mark Zoback and his colleagues have developed a tool that does just that, but in order for it to work, faults need to be well mapped, which is not often the case.
Zoback is an expert on these aquifers, and he is concerned about the pace and scale of development that is now required. “When you start looking at the numbers, they’re mind-boggling,” he says, pointing out that we need to set up hundreds of large capture facilities in the next decade, yet each one currently takes years to analyze and permit, even when everything is going well.
He describes the Gulf Coast Sequestration project in Louisiana. A private family there owns lots of acreage, they have good seismic data and a number of drilled wells (exploratory), and a competent group of former oil and gas professionals is doing the analysis. “They are working their way through the permitting process. Everything is going well. You might call this an easy case, but it’s been four years and they are still a couple of years away.”
I asked if we could set up the wells with less analysis and then cut back the injections if there seems to be a problem, as they have done in Oklahoma and Texas. “No, no, no. Permitting and regulation is critical. Many of the sites will be okay, but without a comprehensive analysis, by the time you find out there’s a problem, you’ve spent tens or hundreds of millions of dollars. There are jobs on the line, economic implications.”
Zoback continues: “I don’t want to sound like I think the end of the world is coming. But if we don’t do it right, it will be like fracking. Everybody hates fracking. We don’t want that to happen with CO2 storage…. Saline aquifers are great. We need to characterize them and pick the right sites, and they will work in the long term. We need to remember, though, that a dot on a map showing a good location can represent a decade of work. The thing that colors my perspective on this whole topic is, how do we get moving in the next ten years?”
He recommends we look more closely at using depleted oil and gas wells. “Things that need to be done have already been done. You start with a lot more knowledge.” He added that many of them have reduced pressure because of the extraction, and so can easily support high volume injections. (In others water has flowed in and so injections may have to proceed more slowly.) Yet many people have deep concerns about using these facilities, in part because the oil and gas industry would profit from the very problem they spent decades downplaying. Zoback understands that, and adds that these depleted wells are not always in the right place. “There are no abandoned oil fields in New York City, and there are certainly lots of emitters there. This is going to be hard no matter which strategy you look at. We just have to be committed and be open to trying all options. This problem is so difficult, so hard, so big, we should not dig ourselves into any one solution.”
It is a sobering experience to speak with a scientist like Zoback, who knows what is being asked to get to net-zero and understands how difficult it is. I heard the CEO of carbon capture company Svante, Claude Letourneau, saying something similar: “I don't think people in general understand the magnitude of what needs to be done.” I wonder whether, if we all clearly understood just how difficult and even improbable the alternatives are, we would be more active in changing some of the things that are in our control, like reducing our fossil-miles, eating a more plant-based diet, buying less stuff, electrifying our homes, and championing related policies. What do you think? I’d love to hear in the comments.
Notes and References
0. Thank you very much to Stanford Professor Emeritus Mark Zoback for his generous time talking about this topic.
1. There are other ways to store carbon dioxide, for example by weathering rocks on the surface or capturing it in cement. This post focuses on underground storage because it looks to have by far the most capacity.
I should also note that this post is not about how we capture the carbon dioxide in the first place. There are many ways to capture CO2, depending in part on where it's coming from (e.g., a smoke stack at a steel factory, oil refinery, or biomass plant; a machine filtering it from the air; etc). This post assumes that the CO2 can be captured. The question then becomes -- where do we put it?
2. Carbon dioxide that is stored at this depth is “supercritical”, with the low viscosity of a gas but the high density of a liquid. More can fit in less space.
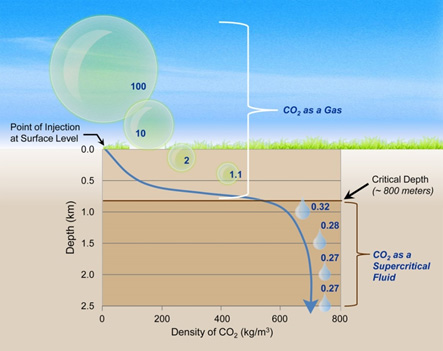
CO2 gets denser at the higher pressures at increasing depths. Source: National Energy Technology Laboratory
3. There are certain types of underground rock formations that mineralize CO2 quickly, such as those made from basalt. Researchers are beginning to explore how quickly and safely CO2 can be injected into such formations, which are relatively common in the Pacific Northwest and the Southeast. The company CarbFix has an active well in Iceland.
4. Some additional difficulties with storing CO2 at scale in underground formations are (a) ownership of those formations, which can span large areas under many properties; and (b) liability over the long-term for leaks. These are discussed some in the National Academy of Sciences report on negative emissions technologies.
5. California is in many ways an easy case for carbon storage. The aquifers are conveniently located, with a large one running down much of the center of the state.
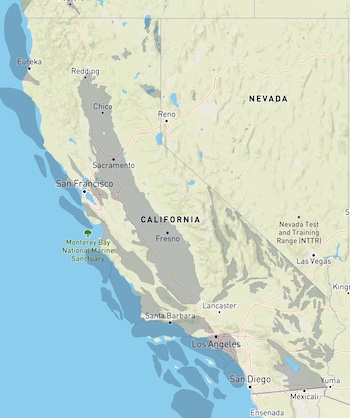
California has sizable saline aquifers beneath much of the state. Source: Department of Energy’s Carbon Storage Atlas (NATCARB)
California’s aquifers are also pretty close to where we are emitting greenhouse gases. That makes the CO2 easier and cheaper to bury.
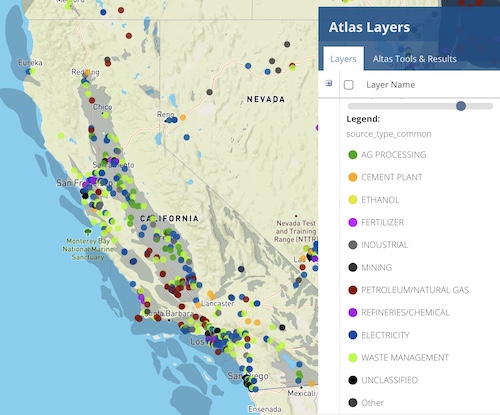
Emission sources shown as colored dots, are generally close to a saline aquifer. Source: Department of Energy’s Carbon Storage Atlas (NATCARB)
The formations are also promising. The lower layer of brittle rocks where the large quakes originate (the “crystalline basement”) is very low and the faults are well mapped. Stanford Professor Sally Benson, an expert in this field, is optimistic about the future of carbon storage in California. She and her colleagues have estimated that we can store about 15% of California’s emissions in underground reservoirs, at a rate of 60 Mt/year, and that we generally have the technology to do so.
They asked stakeholders why more isn’t happening already. One reason is cost. It is expensive to capture and store carbon dioxide, and state incentives are not always available or predictable. Another reason is permitting complexity. Because the process is untested in California, it’s unclear how long the process will take, which makes it harder to plan and to get funding. A 50-page paper from Lawrence Livermore Labs argues that permitting must be sped up if we are to meet our climate goals. But as Zoback suggests, it is hard to speed up permitting in a new area that is scaling up quickly and that regulators are just trying to understand.
6. The National Academy of Sciences has a lot more information on methods of sequestering carbon, and negative emissions more generally, in this report from 2019.
Current Climate Data (March 2022)
Global impacts, US impacts, CO2 metric, Climate dashboard
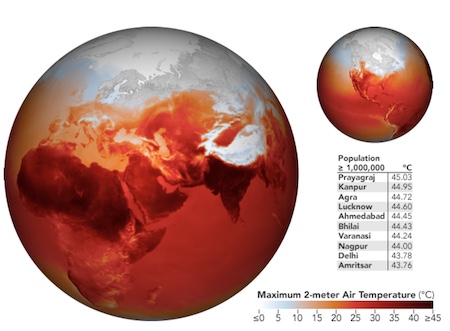
“An intense heat wave in mid- and late April 2022 brought temperatures 4.5 to 8.5°C (8 to 15°F) above normal in east, central, and northwest India—just weeks after the country recorded its hottest March since the country’s meteorological department began keeping records more than 120 years ago.” Source: NASA’s Earth Observatory
Comment Guidelines
I hope that your contributions will be an important part of this blog. To keep the discussion productive, please adhere to these guidelines or your comment may be edited or removed.
- Avoid disrespectful, disparaging, snide, angry, or ad hominem comments.
- Stay fact-based and refer to reputable sources.
- Stay on topic.
- In general, maintain this as a welcoming space for all readers.